
The CHARA Array:
The sharpest eyes in the world
nic scott
Telescope Systems Scientist















X

The Array is capable of resolving details as small as 200 micro-arcseconds, equivalent to the angular size of a nickel (0,20€) coin seen from a distance of 10,000 miles (16,000 km).

Preliminary planning: 1985
engineering studies: 1992
Georgia State $5.6-million 5-telescope array: 1994
NSF capital investment to nearly $6.3-million.
University has provided matching funds in a similar amount.
Ground broken: 1996
Keck Foundation $1.5-million to add a 6th telescope: 1998
First fringes: 1999
The Array achieved starlight fringes on its 331-meter baseline, the longest baseline (by a factor of three) ever achieved by an optical interferometer: 2000



- 0.20 mas at R (650 nm)
- 0.52 mas at H (1.67 μm)
- 0.66 mas at K (2.13 μm)




TelAO




LabAO



MIRX
MYSTIC
SILMARIL
SPICA
CHARIOT
PAVO
VIS BEAMS
METROLOGY
STS/STST
BEAM SAMPLERS
BEAM Reduction
LabAO





Fizeau & Michelson
Brown & Twiss
1850s
1890s
1920 - measured Betelgeuse (with Pease)

1956 - HBT effect, correlation b/t coherent photons
Intensity interferometry



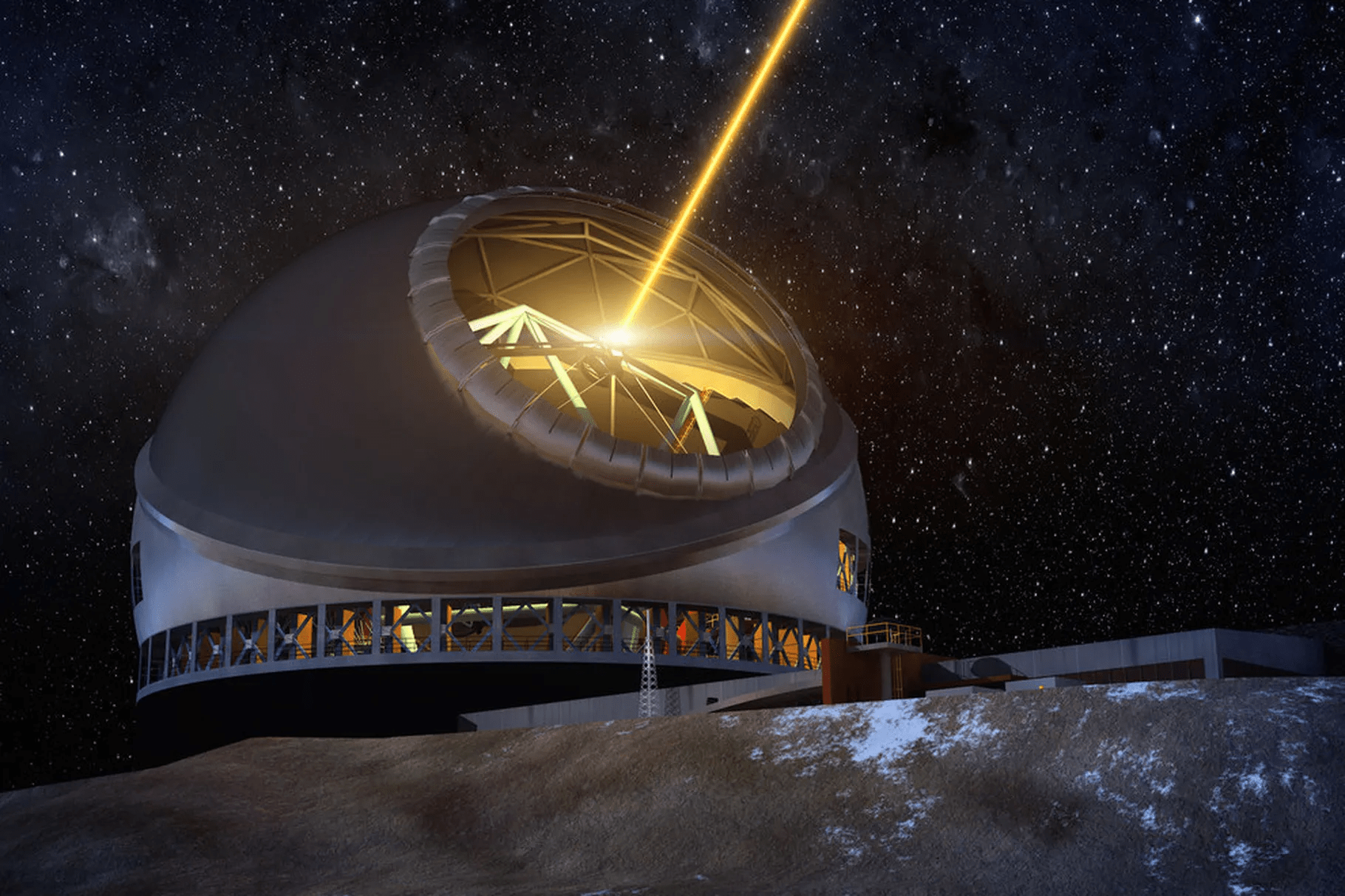
TMT

GMT

ELTs
16x sharper than Hubble ... but CHARA is 17x sharper than ELT




Image credit: ESO





diameter
Limb darkening vs Uniform disk
binarity


Closure phases also yield information about source symmetry
Turbulence in the Earth's atmosphere corrupts the phase of the fringes at optical and near-infrared wavelengths. To recover the phase information, we combine the phases measured in a closed triangle of three telescopes in a way that cancels out the atmospheric turbulence.


Spectrally dispersed fringes produce differential visibilities and differential phases where the visibility and phase of emission lines (like H-alpha or Br-gamma) are measured relative to the stellar continuum. The differential quantities can be used to measure the size and velocity structure of rotating circumstellar disks, outflows, and winds around stars.
Differential Visibilities and Differential Phases
Differential visibilities (left) and differential phases (right) measured for a star surrounded by a circumstellar disk (Meilland et al. 2012, A&A, 538, 110). The drop in the visibility across the emission lines indicate that the disk is more resolved than the stellar continuum. The double-peaked profile corresponds to the blue and red shifted sides of the rotating disk. The S-shaped profile in the differential phase shows a shift in the photo-center across the wavelength channels.


Fringe spacing
Fringe orientation
Fringe Depth (contrast)
Binary separation
Binary position angle
Binary magnitude difference
(delta mag)
relation semimajor-axis (a) and the period (T)
masses of objects
wide binary
close binary
vs
:
:
vs
:
use this to calibrate Mass-Luminosity relationship for single stars






VLTI

up to 140m



up to 331m
the CHARA Array
Spatial resolution
• 0.20 mas at R (650 nm)
• 0.52 mas at H (1.67 μm)
• 0.66 mas at K (2.13 μm)
34 to 331m
- 15 baselines
- 10 closure triangles


1100m
600m
~17m
S3
S4
W5
Max spatial resolution
• 0.06 mas at R (650 nm)
• 0.16 mas at H (1.67 μm)
• 0.20 mas at K (2.13 μm)
17 to 1100m
- 36 possible baselines: array + CMAP [6+3] (15 simultaneous)


The full Michelson Array would offer
12 total positions, creating 66 possible baselines.
CMAP
(CHARA Michelson Array Pathfinder)

Mobile Telescope Transport (TR116)
Ligon et al. 2022







S4

S3


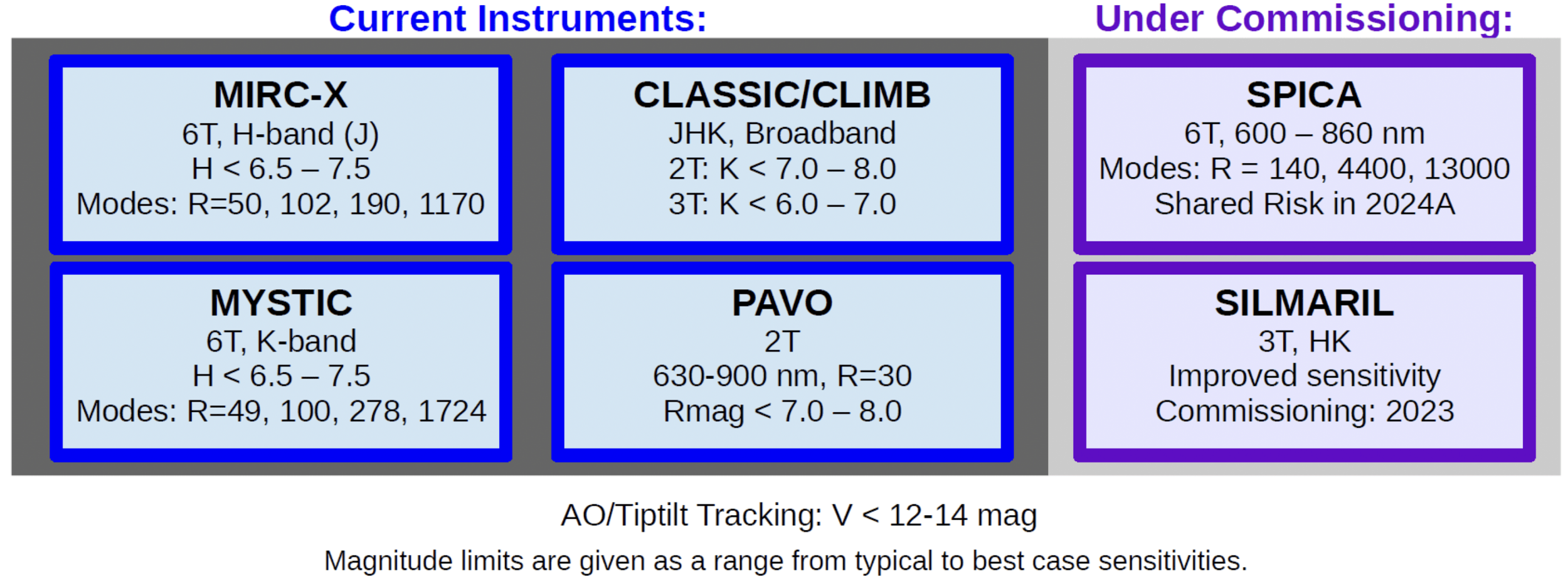



Lanthermann et al. 2022
SILMARIL
MIRCX
MIRC-X disperses light across several spectral channels and operates in the J and H-bands. MYSTIC operates in the K-band. MIRC-X/MYSTIC can be used simultaneously to create images of stellar surfaces and circumstellar disks. The precision closure phases are well suited to detecting faint binary companions.
Three spectral modes are currently available in the H-band: prism R=50 (8 spectral channels), prism R=102, and grism R=190. A higher resolution grism with R=1170 is available (with approval)



STS and STST
Anugu 2020
MYSTIC
MYSTIC, the Michigan Young STar Imager at CHARA is a K-band, cryogenic, 6-beam combiner. All-in-One 6T or high sensitivity 4T gravity chip
A 4-telescope mode for MYSTIC using an integrated optics component designed for the VLTI-GRAVITY experiment is under development and will provide better sensitivity for the faintest targets.
MYSTIC is available in the K-band using a low-resolution R=49 prism. Additional spectra modes (prism R=22, grism R=278, grism R=981, grism R=1724)



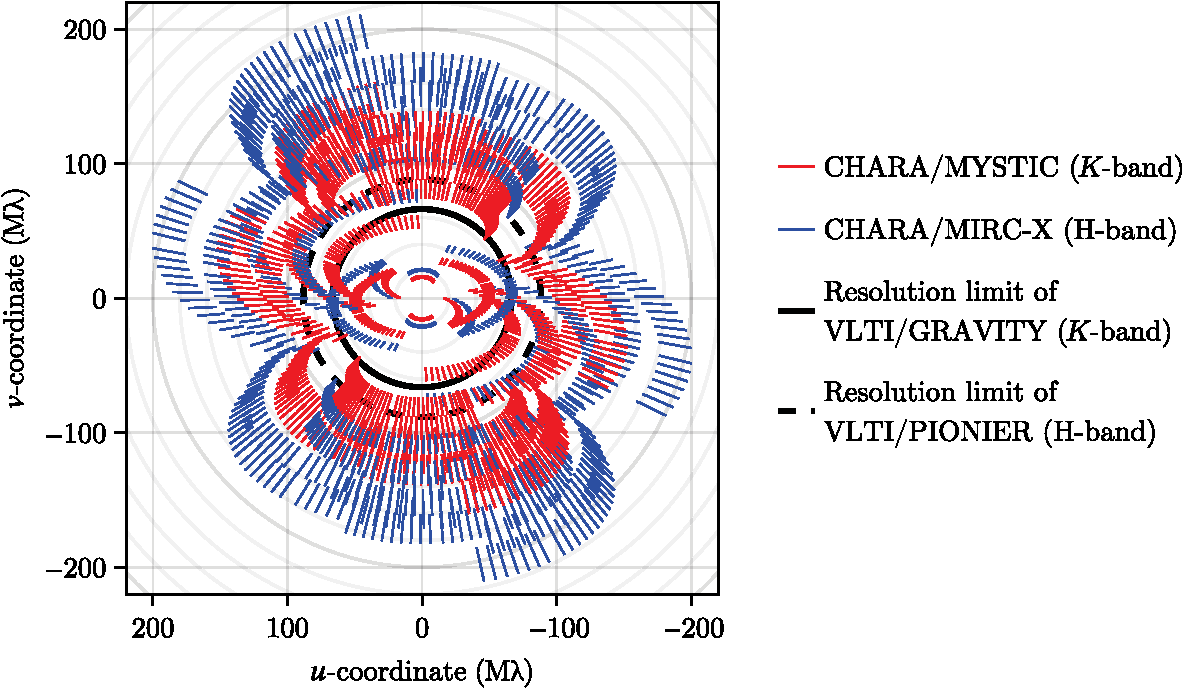
Setterholm et al. 2023

Pannetier et al. 2021
SPICA
(Stellar Parameters and Imaging with a Cophased Array)

SPICA-FT H-band 6-beam ABCD combiner by VLC Photonics, inspired by GRAVITY
low-resolution mode uses MIRC-X for fringe-tracking
The goal of the SPICA project is to provide a large and homogeneous set of stellar parameters across the HR-diagram. The survey aims to measure the angular diameters of 1000 stars.
Low-resolution spectrograph for measuring precise angular diameters (R=150, 50 channels over 650–950 nm).
For a sub-sample of bright stars, medium (R=4300) and high (R=13,200) spectral resolution modes will be available for spectral imaging of stellar surfaces and environments and kinematic studies.

spectrograph

Currently have two injection stages
CHARIOT
(CHARA Array Integrated Optics Testbed)
collaboration with Leibniz Institute for Astrophysics Potsdam (AIP)
ULI optics for JHK bands
(Siliprandi, Labadie, Madhav, Dinkelaker, Thompson, Benoît)
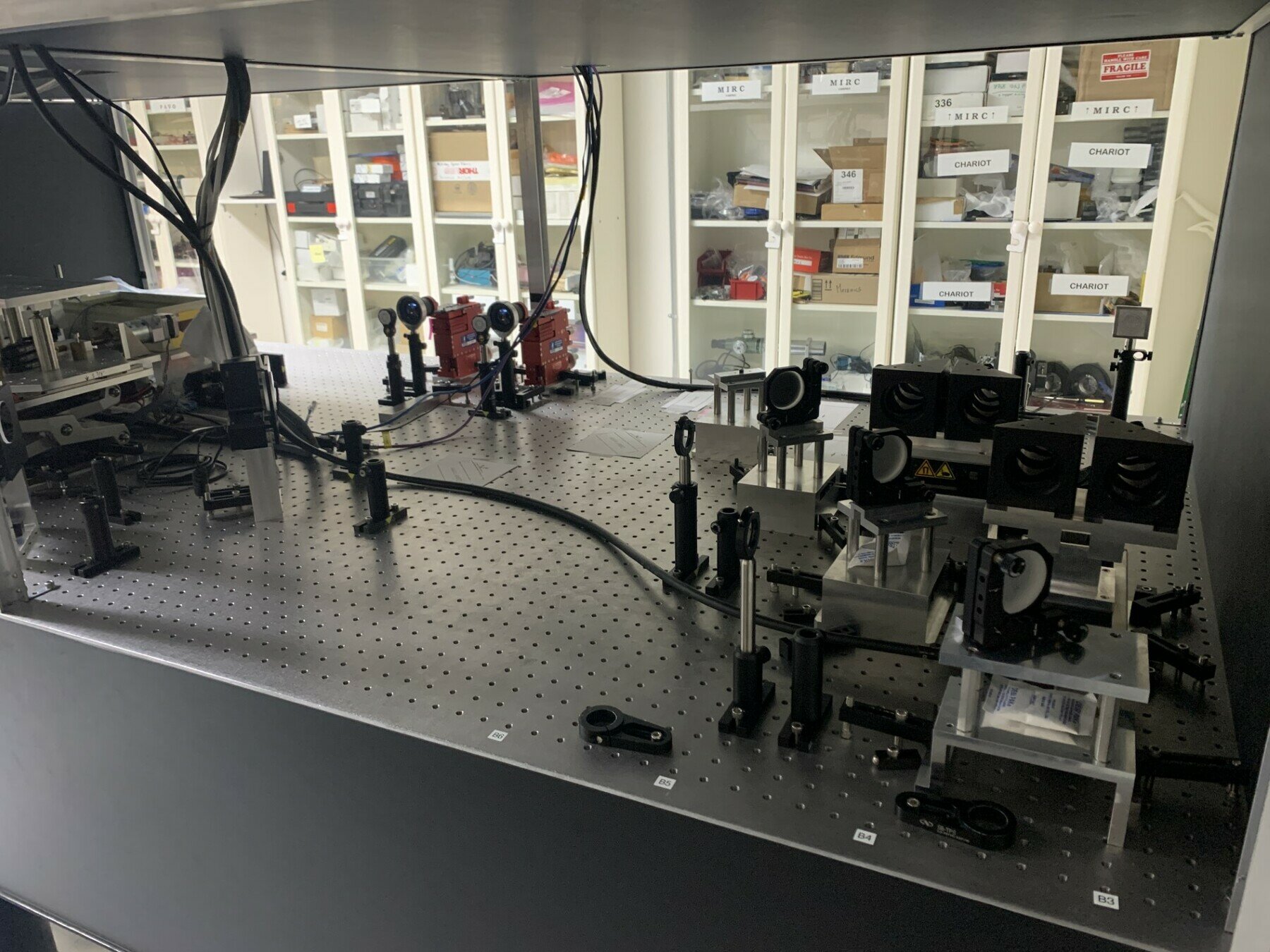

science drivers
- multiple star systems
- time domain astronomy
- YSOs/planetary formation
- evolved stars
- starspots/surface imaging
- exozodis
- AGN
- HWO/PLATO
- exoplanet hosts
There are some 250 known exoplanets with host stars accessible to CHARA (Dec>-30 deg, V < 7). The new baselines will enable resolution of solar-like stars out to about 70pc in H-band. Numerical simulations of the transiting hot-Jupiter in HD189773 indicate that the shape of the silhouette of the planet can be measured in long baseline observations made during transits.
The Gaia orbits give the center-of-light motion of unresolved binaries, and a single resolved CHARA observation is sufficient to determine the full orbit and masses. High angular resolution observations also reveal how interacting stars are transformed by mass exchange.



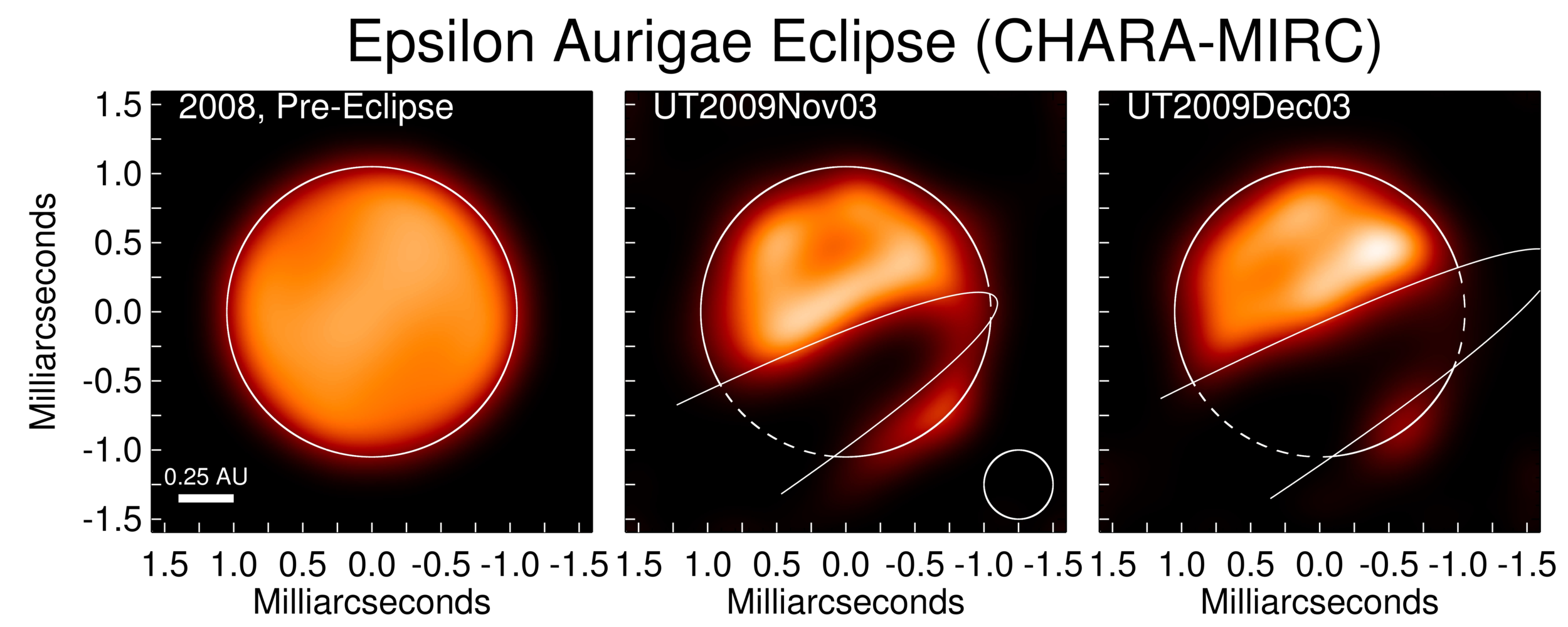


Spotted magnetic stars
Interacting binaries

Expansion curve of Nova Del 2013.


Regulus -- Che et al. 2011, ApJ, 732, 68
Rasalhague -- Zhao et al. 2009, ApJ, 701, 209
Altair -- Monnier et al. 2007, Science, 317, 324
Alderamin -- Zhao et al. 2009, ApJ, 701, 209
Beta Cas -- Che et al. 2011, ApJ, 732, 68



Be stars


~50% of the stars you see are binaries
~25% are triples
-on avg there's an exoplanet per star
~10% of Sun-like stars likely host rocky exoplanets, more for lower-mass stars
-40-50% of Kepler/K2/TESS objects of interest may have companions
-biases occurrence rates & underestimates exoplanet radii by 50%


NGC 4151
Early results from CHARA already show that the innermost dusty region in NGC 4151 is aligned perpendicularly to the jet axis.






future plans
- Telescope dichroic replacement
- more automated alignment and tracking
- new/upgraded labAO system
- TEMA replacement
- new telescope drives, cylinder drives
- fibers to all telescopes (PM fused silica, 1550nm H-band 1st, 1350 nm – 1470 nm metrology)
- nuller "Achromatic photonic tricouplers for application in nulling interferometry" - Martinod et al. 2021
- W5/Channel 13 site (1100m)
- 0.16 mas at H, 65 μas at R
- double-pass delay (90m tracking delay)
-
Narcissus Mirror for SILMARIL
- The Michelson Array
- 2m central telescope?
-
up to 300 nights over three years of open access time via (NOIRLab).
-
Snapshot Imaging Mode to encourage new investigations.



bonus slides


The Rayleigh criterion and the tyranny of the atmosphere


but in atmosphere, turbulent cells limit resolution to
Labeyrie


a method to obtain diffraction-limited resolution across the full aperture of a large telescope
long exposure
speckles blur
produce Airy pattern
true images are impossible, only centrosymmetric objects can be reconstructed, but later work fixed this (mostly)
speckle pattern is the Fourier transform of telescope pupil
autocorrelation of speckles
(in Fourier space)
modulus
(time-averaged intensity)
-
Explore the apparent variability of known exozodis
- long-term monitoring
- clues to source and formation of the dust
-
Expand strong exozodi sample
- leveraging LBTI and prior surveys
- from ~100 \(\rightarrow\) ~1000 objects
- Use spectral dispersion to resolve the thermal/scattered dilemma
- Risk mitigation for coronagraphy/starshade missions
-
Target selection and characterization for mid/large missions (HWO, Plato, etc)
- exozodis likely to be dominant noise source
- Precision diameters and fundamental astrophysics

following slides stolen from EXOPAG 23:
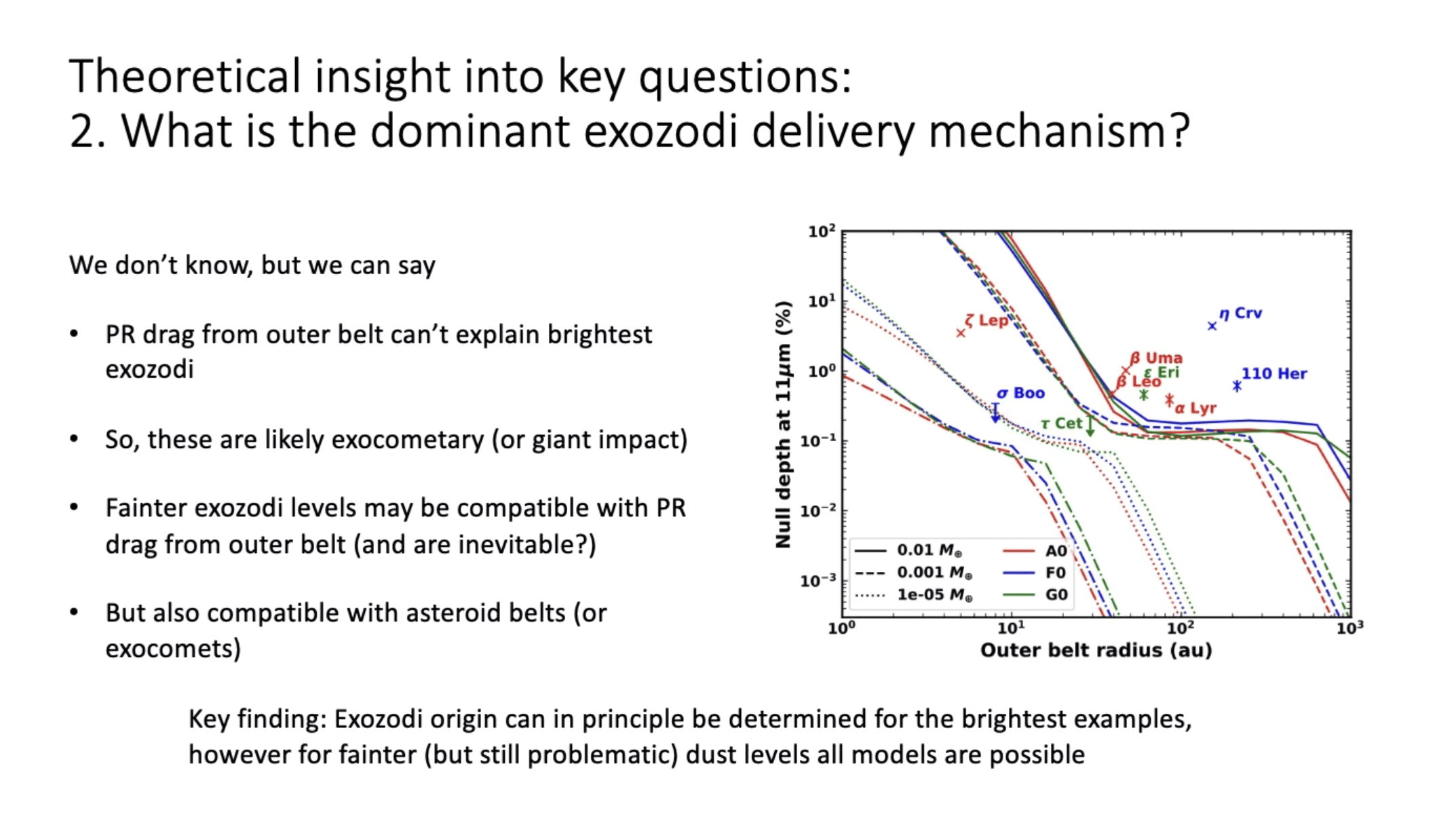





-
Planewave:
- 2-3 yrs until a 2 meter
- $2.5 million ea
- > 2 magnitudes deeper
-
move existing telescopes to CMAP sites?
- 1m outriggers?
- 6x2m + 4x1m array? 45 baselines!
-
JWST plenary talk:
- “imaging interferometers are coming online”
-
How to get more hands at the Array/instrumentation interest
- REU options
- Shadow-a-Scientist programs
- Collaborations/Partners
-
Funding
- NSF wants astronomers to compete for MSIPs with MSRI funds
- Workshops on centers of excellence, partnerships with industry (TIP)
- Could chara get solar panel funding?
- Grow local and state politician interest/investment?
Some thoughts from AAS 241













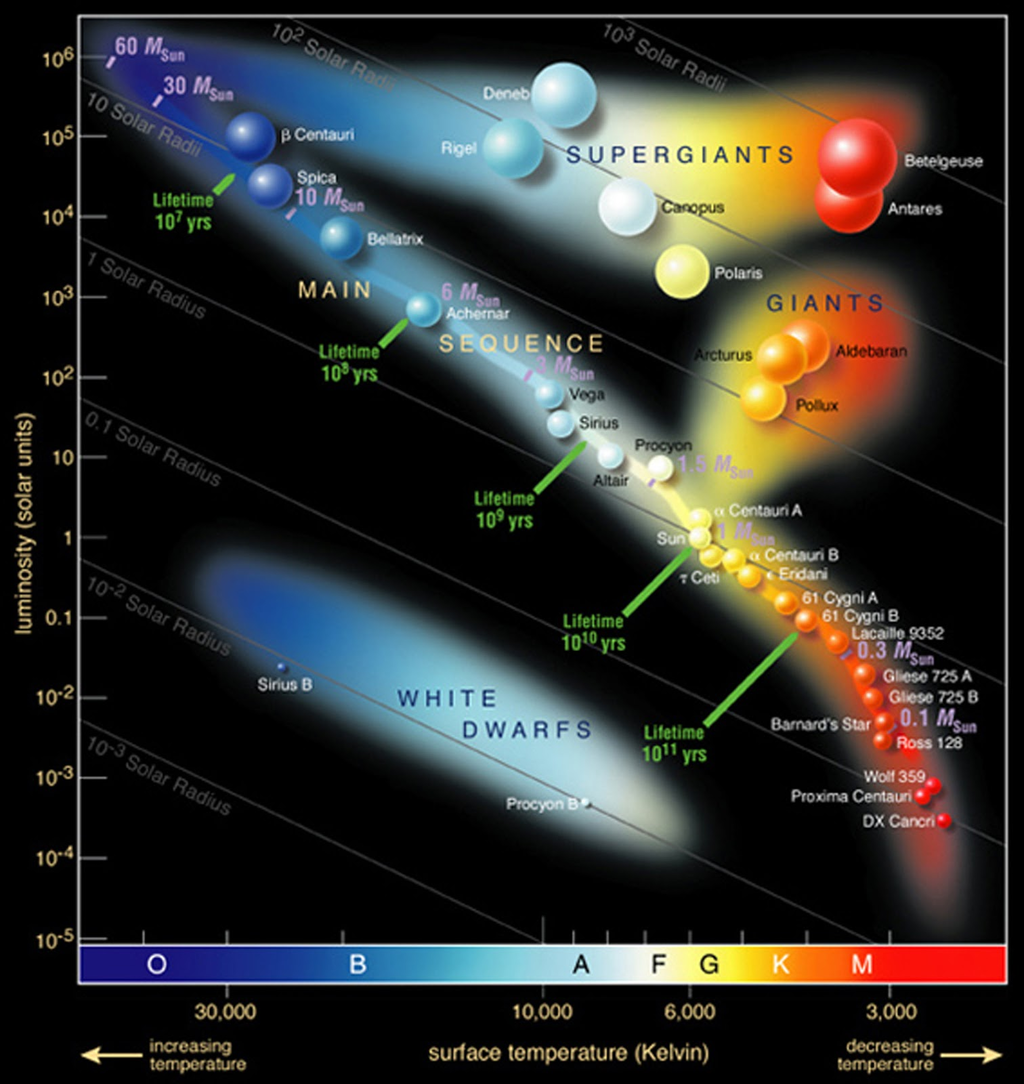

<0.08
0.08-0.4
0.4-8
>8
>20
>3
1.4-3
>1.4
Solar Masses



Oxygen

- get transmission spectrum of the atmosphere
- measure different radii at different wavelegnths to give clues to composition


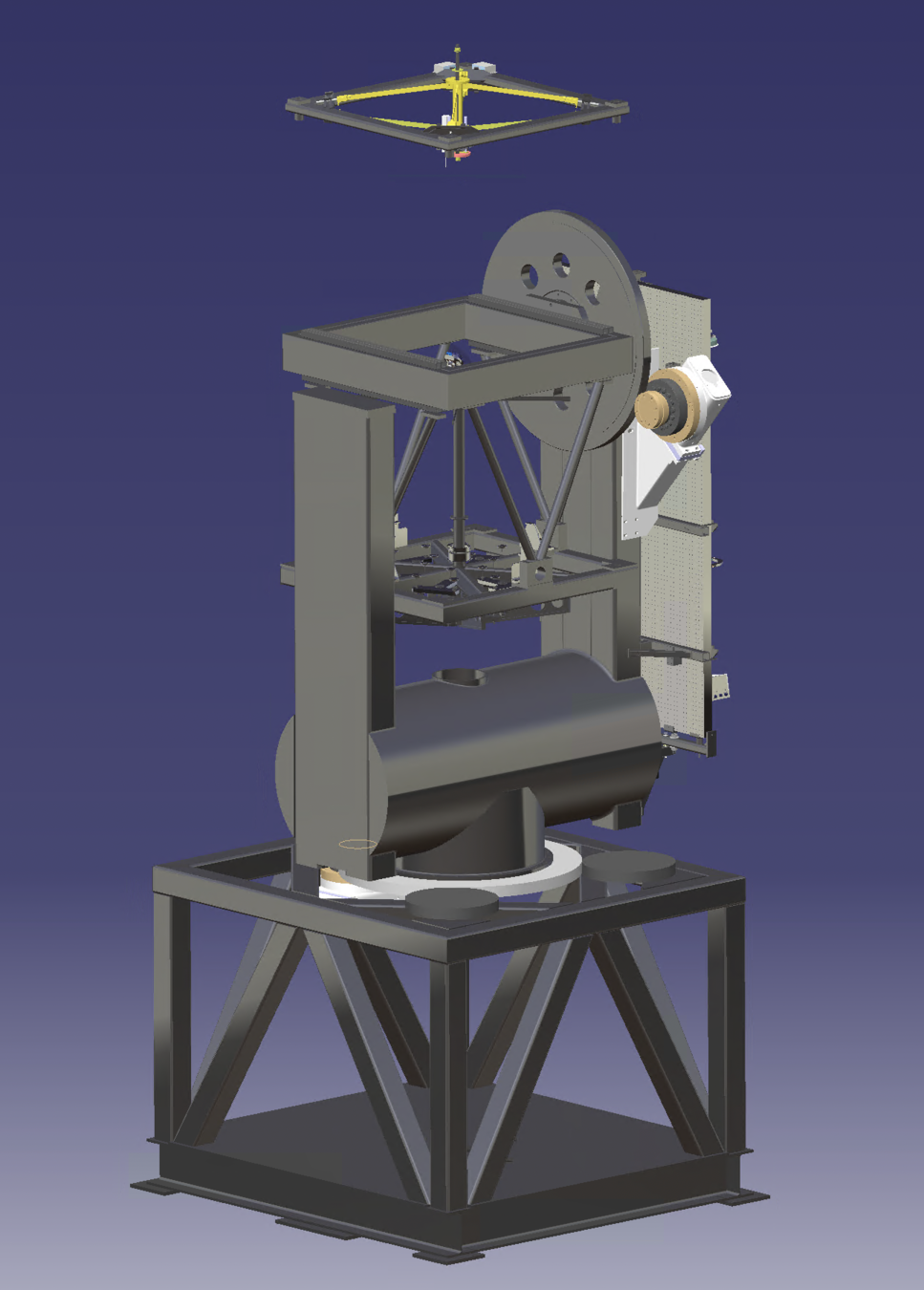


CHARIOT
(CHARA Array Integrated Optics Testbed)

collaboration with Leibniz Institute for Astrophysics Potsdam (AIP)
ULI optics for JHK bands
(Siliprandi, Labadie, Madhav, Dinkelaker, Thompson, Benoît)


Coupling ratio evolutions of (input coupling waveguide) and R2(λ) in blue diamonds of a writing beam combiner characterized by coupling input light in the two external PT1 and PT2 inputs to obtain two experimental measurements of the 3 dB asymmetric directional coupler (a, b) and one for each photometric asymmetric Tap directional couplers, as Tap 1 (c) and Tap 2 (d)

Siliprandi et al. 2022

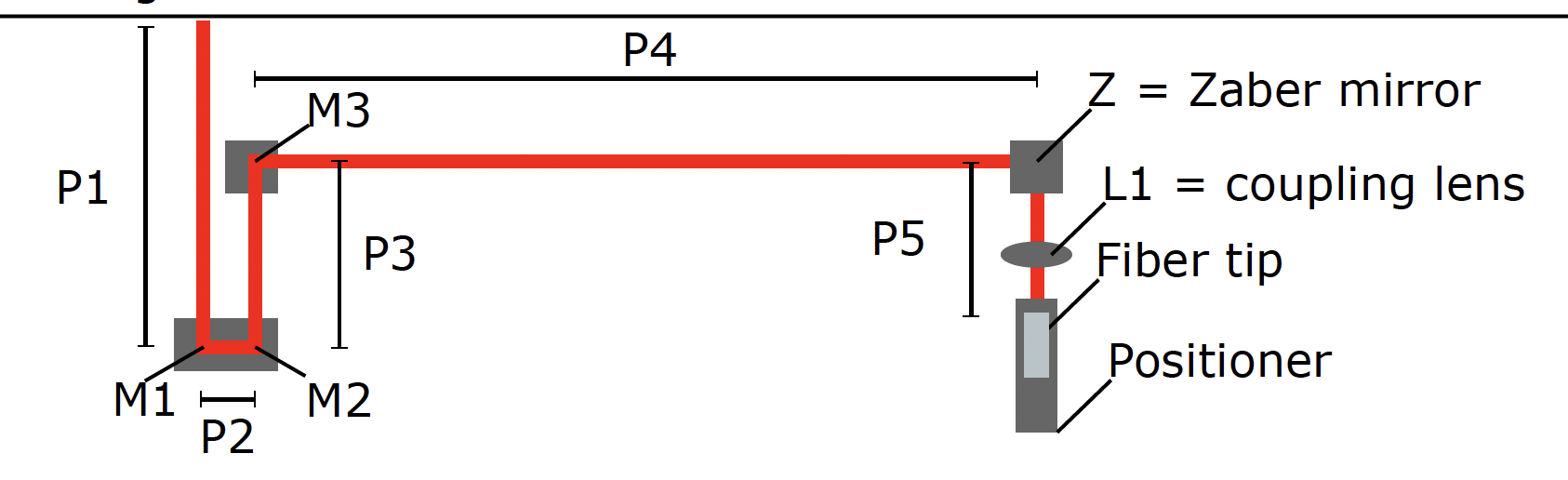





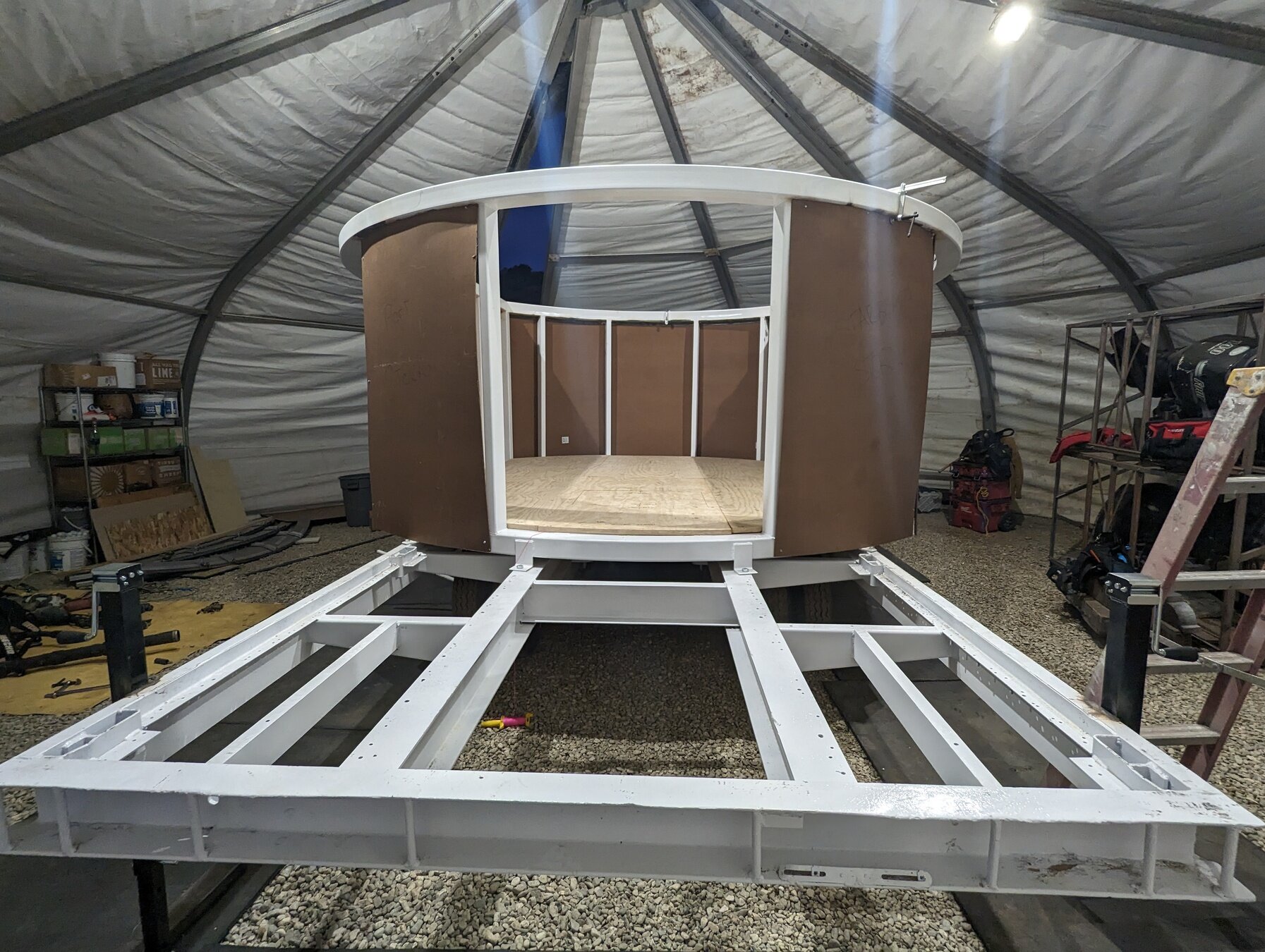













All we have to measure is light from a distant star.....so how can we find out so much about them?
flux
spectra
position
wavefront
What can we measure from light?
Characteristics
-
Intensity ("flux")
-
photometry
- magnitudes
- transits
-
photometry
-
Wavelength ("color")
-
spectrum
- radial velocity
- composition
- incandescence
- emission
- absorption
-
spectrum
- Interference
- Polarization
- Timing




Old but interesting. Perhaps a reader is ready to modernize the list?? I believe this is from Shapley's book "Beyond the Observatory."
******************
“Thirty Deductions from a Glimmer of Starlight”
Harlow Shapley
… let us return to a consideration of what modern astronomers can find out about a single star. I shall list here thirty facts that we can now deduce from appropriate studies of a single star image. The first eighteen of these facts can be discovered about any star.
1. The position in the sky with reference to other stars.
2. The apparent magnitude (brightness) with reference to stellar or artificial standards.
3. The color index (found by comparing the brightness in various spectrum intervals—that is, measuring the color tint: reddish, yellowish, greenish, or bluish).
4. The variability in light; it may be zero.
5. The spectral class in two dimensions.
6. The variability, if any, in spectral class.
7. The chemical composition of the stellar atmosphere and the consequent nature of the atomic transformations that maintain the radiation.
8. The approximate age.
9. Whether it is a single or double (found in various ways).
10. The existence and strength of its magnetic field.
11. The involvement with interstellar nebulosity.
12. The speed of rotation.
13. The tilt of the rotational axis.
14. The speed in the line of sight, and variations, regular or irregular in that speed.
15. The cross motion—measurable only if the distance of the star is small or the speed is great.
16. The surface temperature.
17. The total luminosity (candle power).
18. The diameter.
The next eight facts can also be learned if the star is an eclipsing binary—a double star whose light varies because the two members of the system periodically eclipse each other.
1. The mean density of the two components.
2. The period of revolution.
3. The geometry of the eclipse—and whether it is total or partial.
4. The degree of darkening at the lib.
5. The ratio of the sizes of the two components.
6. The eccentricity of the relative orbit.
7. The inclination of the orbital plane.
8. The approximate distance.
And about a Cepheid variable—a star that varies in light periodically because of pulsations—four additional facts can be found.
1. The shape of the light curve.
2. The period of pulsation.
3. The population of membership.
4. The approximate distance.
magnetic fields
For many stars, we can now add: the frequencies and amplitudes of its oscillations (asteroseismology)
presence of planets
Let's consider the state-of-the-art in "single star images" - resolved interferometric imaging in the optical can yield:
* Stellar shapes - eg. particularly oblateness for rapid rotators
* Surface brightness distribution - including star spots, stellar limb darkening, gravity darkening
* Interior temperature structure of the upper atmosphere (by inverting the limb darkening)
* For rapid rotators, gravity darkening can directly indicate the degree of convective versus radiative energy transfer
* And the star spots can make Bryan happy by giving insight into magnetic fields 😎
CHARA intro
By Nic Scott
CHARA intro
- 144